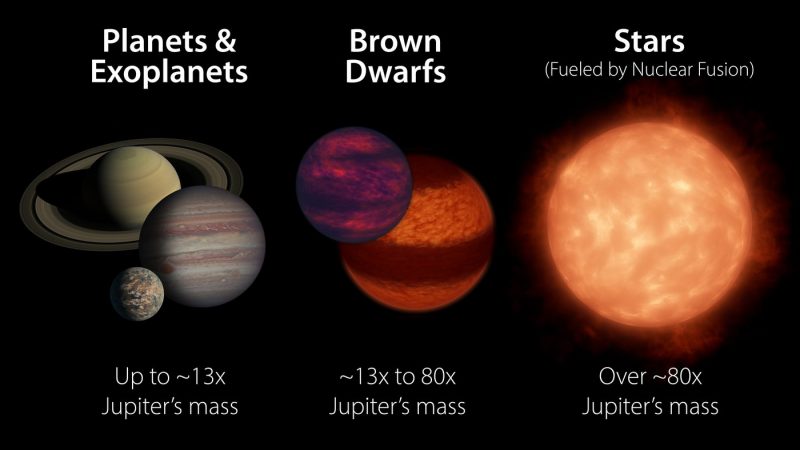
Brown dwarfs are determined by their mass
The amount of mass a star is born with is what determines its fate. Stars are objects born with large masses, and therefore strong self-gravity. As a result, the star squeezes in on itself, creating high internal temperatures. The high temperatures spark thermonuclear fusion reactions, which enable stars to shine.
Planets have much smaller masses and consequently weaker gravity with no internal fusion. So planets shine by the light reflected from their stars.
Brown dwarfs fall somewhere between the masses of giant planets like Jupiter and the smallest stars. We could speak of brown dwarf masses as fractions of our sun’s mass. But, astronomers use Jupiter’s mass as a standard measure for them.
A value of 13 Jupiter-masses is considered to be the upper limit for gas giant planets. If the mass of the gas planet is larger than 13 Jupiter-masses, and less than 80 Jupiter-masses, a thermonuclear burning (fusion) of deuterium can occur in the object’s interior. Deuterium is another name for heavy hydrogen. Heavy hydrogen is hydrogen with a neutron attached to the proton in the nucleus of the atom (rather than only a proton alone).
Also, a value of greater than 80 Jupiter-masses is the lower limit for burning normal hydrogen, the process that causes stars to shine. And the process that enables an object to qualify as a full-fledged star. So brown dwarfs do not have enough mass to fuse normal hydrogen like a regular star. In fact, some call a brown dwarf a failed star.
What is a star?
A star is a large collection of dust and gas that has condensed from a disturbance of a primordial cloud. Various mechanisms can cause the disturbance. For example, the shock wave from a distant supernova – an exploding star – might disturb a primordial cloud in space, centuries or millennia later and many light-years away. Then the cloud loses its uniformity, and areas with slightly higher density (and thus more gravity) start to attract lighter molecules.
As matter keeps clumping tighter and tighter due to its now-increasing gravity, it gets hotter and hotter. Eventually the matter reaches a critical mass; the protostar starts to fuse deuterium with regular hydrogen, making helium-3 molecules. This occurs at a low temperature (slightly less than 1,000,000 degrees Kelvin or 1,800,000 Fahrenheit).
Then stars start to form
In most cases, what’s left is a single major accretion that forms a hydrogen-fusion-powered star. Also, it’s possible that, in dense clouds, multiple stars can form. And thus we have double-star systems (called a binary system) and triple stars (trinary) and quadruple stars (quaternary), respectively (click each number for examples).
Indeed, there are examples of more complex systems with five, six, and seven stars, called quintuple (quintenary), sextuple (sextenary), and septuple (septenary), respectively (click each number for examples). These can fall into orbits around each other that (although very complex) can still be stable enough to allow planetary formation.
And then fusion begins
At the point where fusion begins, we can describe a star differently. Now the star is an object in perfect balance (however temporarily) between the outward-pushing force caused by the fusion reactions in its core, and inward-pushing force of its own self-gravity. Gravity wants to crush a star further, but fusion prevents that from happening. Fusion wants to expand the star, but gravity won’t let it. The result is a fine balance between pressure and gravity in the star.
If deuterium fusion didn’t take place in protostars, there would be very few stars in the universe with more than three times the mass of our sun. That’s because – if hydrogen fusion started as soon as the mass and temperature were high enough – the star wouldn’t yet have enough mass for its own self-gravity to resist the outward-pushing pressure of the hydrogen fusion reactions. So the star would expand, and this expansion would cause its internal temperature to drop. And this would slow and ultimately end the hydrogen fusion reactions stars require to shine.
Deuterium fusion keeps a star cold enough to allow time for the star to accumulate sufficient mass. So then when hydrogen fusion starts (around 13,000,000 degrees K or 23,000,000 F), it can continue. By that time, the star is dense enough to have enough self-gravity to resist expansion, and temperatures stay high in its interior. Only more massive stars begin fusing hydrogen, but brown dwarfs never gain enough mass to begin fusing hydrogen and become stars.
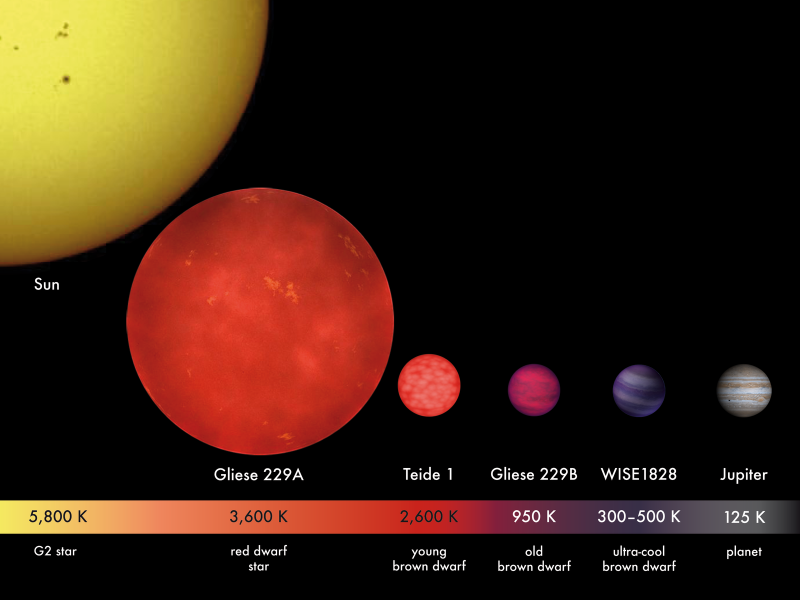
Inside a stellar nursery
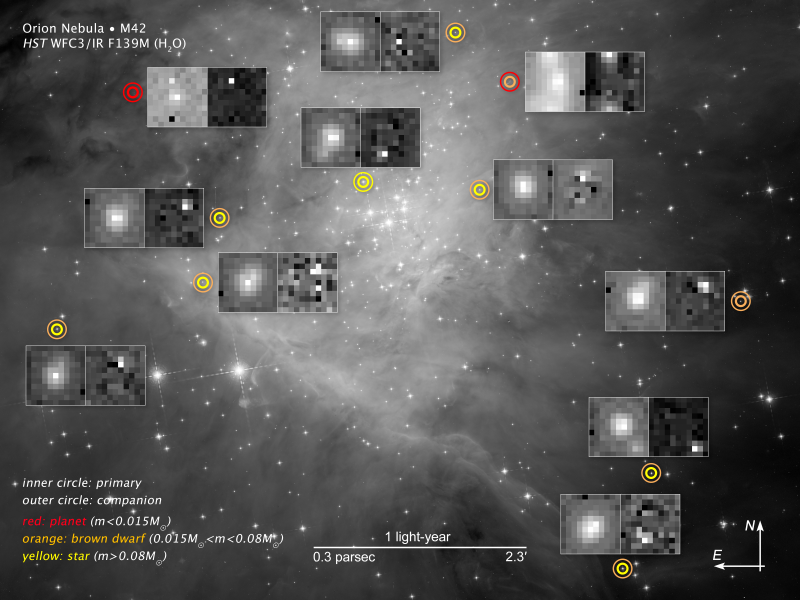
How planets form
After stellar formation and the beginning of hydrogen fusion, a solar wind sweeps the remaining gas out of the system. Also, there will be several minor accretions too bulky to be pushed away by the solar wind. In fact, they will fall inward towards the star.
Since everything in the universe has angular momentum – in other words, since the primordial cloud is rotating or spinning – particles in the initial cloud collecting to form the star will have a tendency to fall in toward the star in a long spiral path. This increases their fall time and thus angular speed. So this is why planets end up themselves rotating (spinning) and orbiting their stars generally all in the same direction.
Due to collisions and mutual attractions altering the orbits of the newly forming protoplanets, many will reach an equilibrium point and settle into a stable orbit. These will eventually become true planets – either rocky worlds like Earth or Mars, or gas giants like Jupiter or Saturn – by accreting the remaining small leftovers of the original primordial cloud via their own gravity.
The difference between stars and planets
Stars form from the collapse of gas and dust in a primordial cloud. Consequently, they have a relatively low amount of what astronomers call metals. By the way, astronomers refer to any element heavier than hydrogen and helium as metals.
First, the star collects most of the gaseous material in the primordial cloud. And then, its planets form by accreting the leftovers. Planets form with much, much less mass than stars, and thus have much weaker gravity. The lighter elements like hydrogen and helium – so common in stars – tend to escape a planet’s weaker gravitational pull. Thus – relative to stars – planets have high metal content. Planets typically orbit stars. By astronomers’ most recent definition of the word planet, they clear their own orbits of debris.
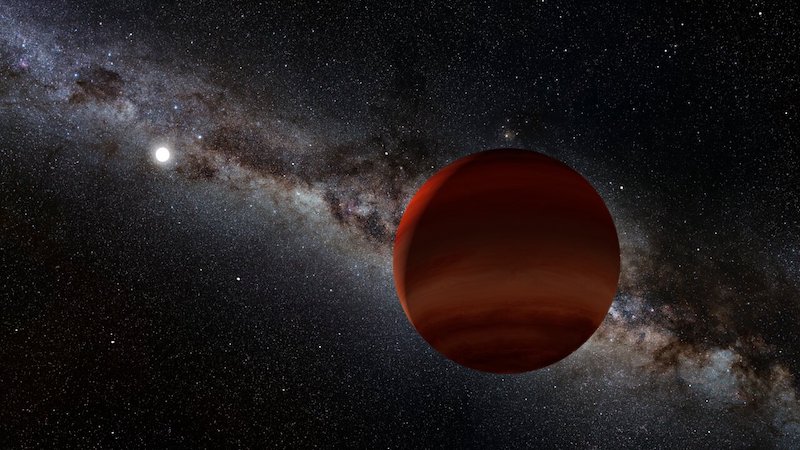
Where does that leave brown dwarfs?
Brown dwarfs accumulate material like a star, not like a planet. They condense from a gaseous cloud and are higher in mass than planets and so have stronger gravity. Thus, they hold onto their lighter elements (hydrogen and helium) more effectively than planets and have a relatively low metal content. Their only failing feature is that they didn’t collect enough material to begin normal stellar fusion. Although, they can sustain deuterium fusion until the deuterium is gone, which is essential to stellar formation with larger masses, as explained earlier.
Brown dwarfs have been found orbiting other suns at distances of 1,000 astronomical units (AU) or more. One AU = one Earth-sun distance. However, not all brown dwarfs orbit far from their stars; some have been found orbiting at closer distances, and a few rogue brown dwarfs have been spotted not orbiting any star. Of course, these rogue brown dwarfs are tough to find!
As a comparison, of the known planets in our own solar system, Neptune is the major planet orbiting farthest from our sun at 30 AU.
So brown dwarfs are not planets, and they are not stars. They are failed stars, not massive enough to power hydrogen fusion reactions. Thus, they get their own classification.
Why call them brown dwarfs?
Astronomer Shiv S. Kumar, speculated brown dwarfs existed in the 1960’s. He originally called them black dwarfs. He pictured them as dark substellar objects floating freely in space that were not massive enough to sustain hydrogen fusion.
Then SETI researcher Jill Tarter coined the name brown dwarf in her Ph.D. dissertation. She was looking to define an upper limit to the maximum mass an object could possess before beginning hydrogen fusion, and thus becoming a full-fledged star.
Stars are clearly not “brown” and many such objects are in the temperature range of 300 to 500 Kelvin (80 to 440 F), so they only radiate in the infrared portion of the electromagnetic spectrum. Since black dwarf was already taken for describing objects at the end point in stellar evolution (or cold white dwarfs) – and red dwarf is the name for small, cool stars – brown must have seemed an appropriate compromise.
Bottom line: Brown dwarfs are objects with a mass that range between the heaviest gas giant planets and the lightest stars. This makes them distinct enough to qualify for their own classification. So they are typically defined as a body lying in the range of greater than 13 and less than 80 Jupiter-masses.
Read more: Webb detects sand clouds for brown dwarf
The post What are brown dwarfs? first appeared on EarthSky.
from EarthSky https://ift.tt/VTLx8oG
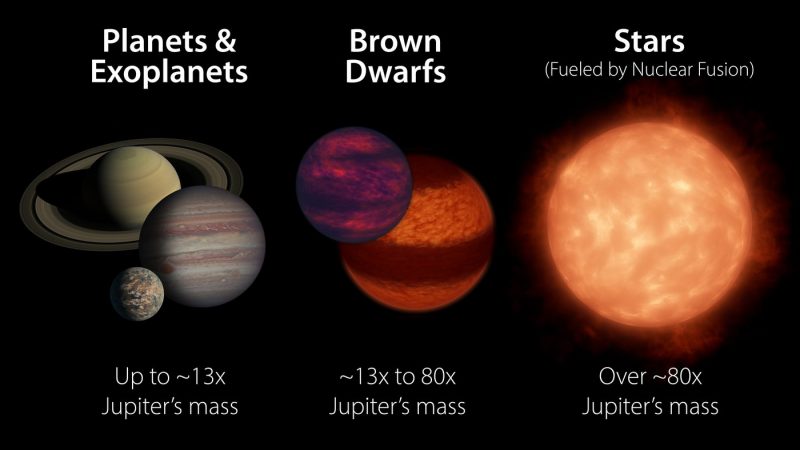
Brown dwarfs are determined by their mass
The amount of mass a star is born with is what determines its fate. Stars are objects born with large masses, and therefore strong self-gravity. As a result, the star squeezes in on itself, creating high internal temperatures. The high temperatures spark thermonuclear fusion reactions, which enable stars to shine.
Planets have much smaller masses and consequently weaker gravity with no internal fusion. So planets shine by the light reflected from their stars.
Brown dwarfs fall somewhere between the masses of giant planets like Jupiter and the smallest stars. We could speak of brown dwarf masses as fractions of our sun’s mass. But, astronomers use Jupiter’s mass as a standard measure for them.
A value of 13 Jupiter-masses is considered to be the upper limit for gas giant planets. If the mass of the gas planet is larger than 13 Jupiter-masses, and less than 80 Jupiter-masses, a thermonuclear burning (fusion) of deuterium can occur in the object’s interior. Deuterium is another name for heavy hydrogen. Heavy hydrogen is hydrogen with a neutron attached to the proton in the nucleus of the atom (rather than only a proton alone).
Also, a value of greater than 80 Jupiter-masses is the lower limit for burning normal hydrogen, the process that causes stars to shine. And the process that enables an object to qualify as a full-fledged star. So brown dwarfs do not have enough mass to fuse normal hydrogen like a regular star. In fact, some call a brown dwarf a failed star.
What is a star?
A star is a large collection of dust and gas that has condensed from a disturbance of a primordial cloud. Various mechanisms can cause the disturbance. For example, the shock wave from a distant supernova – an exploding star – might disturb a primordial cloud in space, centuries or millennia later and many light-years away. Then the cloud loses its uniformity, and areas with slightly higher density (and thus more gravity) start to attract lighter molecules.
As matter keeps clumping tighter and tighter due to its now-increasing gravity, it gets hotter and hotter. Eventually the matter reaches a critical mass; the protostar starts to fuse deuterium with regular hydrogen, making helium-3 molecules. This occurs at a low temperature (slightly less than 1,000,000 degrees Kelvin or 1,800,000 Fahrenheit).
Then stars start to form
In most cases, what’s left is a single major accretion that forms a hydrogen-fusion-powered star. Also, it’s possible that, in dense clouds, multiple stars can form. And thus we have double-star systems (called a binary system) and triple stars (trinary) and quadruple stars (quaternary), respectively (click each number for examples).
Indeed, there are examples of more complex systems with five, six, and seven stars, called quintuple (quintenary), sextuple (sextenary), and septuple (septenary), respectively (click each number for examples). These can fall into orbits around each other that (although very complex) can still be stable enough to allow planetary formation.
And then fusion begins
At the point where fusion begins, we can describe a star differently. Now the star is an object in perfect balance (however temporarily) between the outward-pushing force caused by the fusion reactions in its core, and inward-pushing force of its own self-gravity. Gravity wants to crush a star further, but fusion prevents that from happening. Fusion wants to expand the star, but gravity won’t let it. The result is a fine balance between pressure and gravity in the star.
If deuterium fusion didn’t take place in protostars, there would be very few stars in the universe with more than three times the mass of our sun. That’s because – if hydrogen fusion started as soon as the mass and temperature were high enough – the star wouldn’t yet have enough mass for its own self-gravity to resist the outward-pushing pressure of the hydrogen fusion reactions. So the star would expand, and this expansion would cause its internal temperature to drop. And this would slow and ultimately end the hydrogen fusion reactions stars require to shine.
Deuterium fusion keeps a star cold enough to allow time for the star to accumulate sufficient mass. So then when hydrogen fusion starts (around 13,000,000 degrees K or 23,000,000 F), it can continue. By that time, the star is dense enough to have enough self-gravity to resist expansion, and temperatures stay high in its interior. Only more massive stars begin fusing hydrogen, but brown dwarfs never gain enough mass to begin fusing hydrogen and become stars.
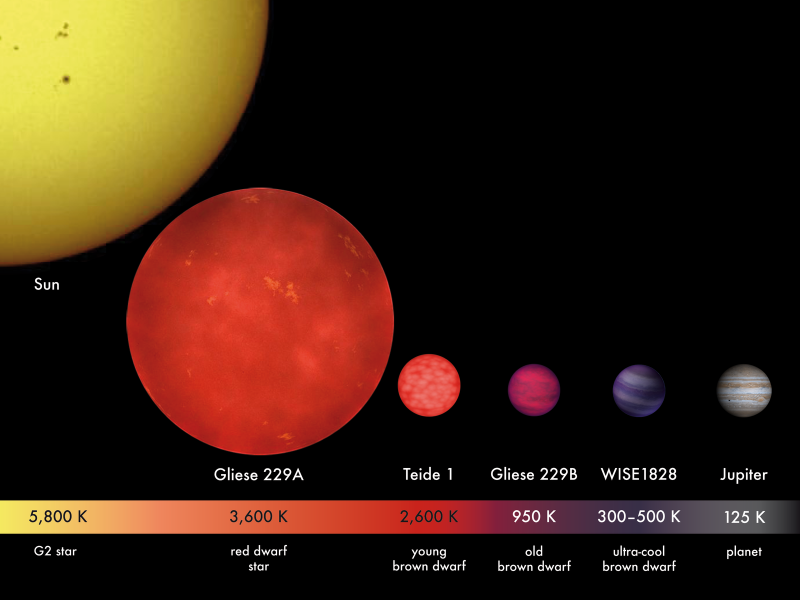
Inside a stellar nursery
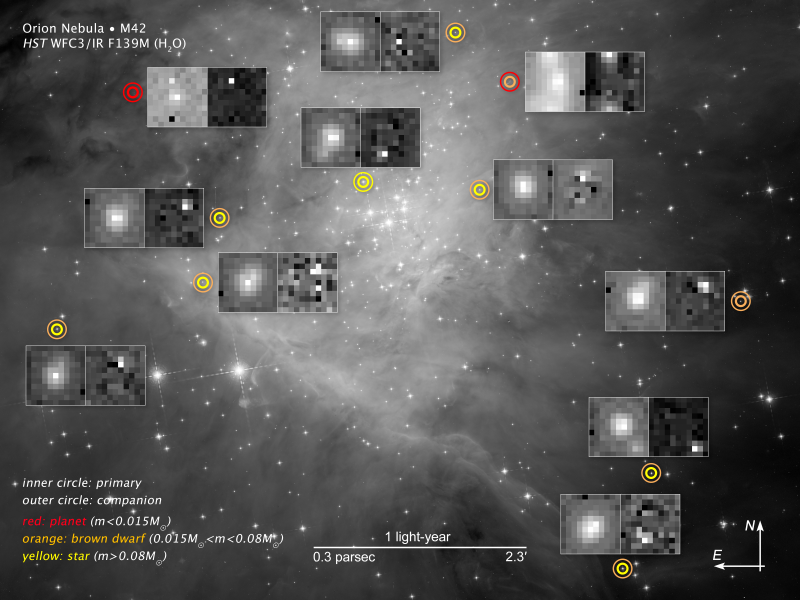
How planets form
After stellar formation and the beginning of hydrogen fusion, a solar wind sweeps the remaining gas out of the system. Also, there will be several minor accretions too bulky to be pushed away by the solar wind. In fact, they will fall inward towards the star.
Since everything in the universe has angular momentum – in other words, since the primordial cloud is rotating or spinning – particles in the initial cloud collecting to form the star will have a tendency to fall in toward the star in a long spiral path. This increases their fall time and thus angular speed. So this is why planets end up themselves rotating (spinning) and orbiting their stars generally all in the same direction.
Due to collisions and mutual attractions altering the orbits of the newly forming protoplanets, many will reach an equilibrium point and settle into a stable orbit. These will eventually become true planets – either rocky worlds like Earth or Mars, or gas giants like Jupiter or Saturn – by accreting the remaining small leftovers of the original primordial cloud via their own gravity.
The difference between stars and planets
Stars form from the collapse of gas and dust in a primordial cloud. Consequently, they have a relatively low amount of what astronomers call metals. By the way, astronomers refer to any element heavier than hydrogen and helium as metals.
First, the star collects most of the gaseous material in the primordial cloud. And then, its planets form by accreting the leftovers. Planets form with much, much less mass than stars, and thus have much weaker gravity. The lighter elements like hydrogen and helium – so common in stars – tend to escape a planet’s weaker gravitational pull. Thus – relative to stars – planets have high metal content. Planets typically orbit stars. By astronomers’ most recent definition of the word planet, they clear their own orbits of debris.
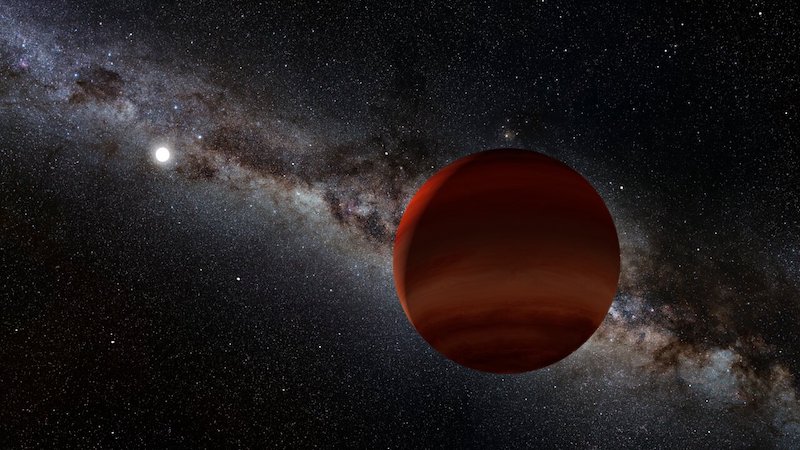
Where does that leave brown dwarfs?
Brown dwarfs accumulate material like a star, not like a planet. They condense from a gaseous cloud and are higher in mass than planets and so have stronger gravity. Thus, they hold onto their lighter elements (hydrogen and helium) more effectively than planets and have a relatively low metal content. Their only failing feature is that they didn’t collect enough material to begin normal stellar fusion. Although, they can sustain deuterium fusion until the deuterium is gone, which is essential to stellar formation with larger masses, as explained earlier.
Brown dwarfs have been found orbiting other suns at distances of 1,000 astronomical units (AU) or more. One AU = one Earth-sun distance. However, not all brown dwarfs orbit far from their stars; some have been found orbiting at closer distances, and a few rogue brown dwarfs have been spotted not orbiting any star. Of course, these rogue brown dwarfs are tough to find!
As a comparison, of the known planets in our own solar system, Neptune is the major planet orbiting farthest from our sun at 30 AU.
So brown dwarfs are not planets, and they are not stars. They are failed stars, not massive enough to power hydrogen fusion reactions. Thus, they get their own classification.
Why call them brown dwarfs?
Astronomer Shiv S. Kumar, speculated brown dwarfs existed in the 1960’s. He originally called them black dwarfs. He pictured them as dark substellar objects floating freely in space that were not massive enough to sustain hydrogen fusion.
Then SETI researcher Jill Tarter coined the name brown dwarf in her Ph.D. dissertation. She was looking to define an upper limit to the maximum mass an object could possess before beginning hydrogen fusion, and thus becoming a full-fledged star.
Stars are clearly not “brown” and many such objects are in the temperature range of 300 to 500 Kelvin (80 to 440 F), so they only radiate in the infrared portion of the electromagnetic spectrum. Since black dwarf was already taken for describing objects at the end point in stellar evolution (or cold white dwarfs) – and red dwarf is the name for small, cool stars – brown must have seemed an appropriate compromise.
Bottom line: Brown dwarfs are objects with a mass that range between the heaviest gas giant planets and the lightest stars. This makes them distinct enough to qualify for their own classification. So they are typically defined as a body lying in the range of greater than 13 and less than 80 Jupiter-masses.
Read more: Webb detects sand clouds for brown dwarf
The post What are brown dwarfs? first appeared on EarthSky.
from EarthSky https://ift.tt/VTLx8oG
Aucun commentaire:
Enregistrer un commentaire