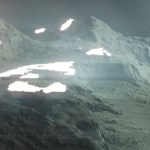

Comet 67P/Churyumov-Gerasimenko as seen by the Rosetta spacecraft on September 22, 2014. Amateur astronomer Jacint Roger Perez, from Spain, processed this image, which is via ESA/Rosetta/MPS for OSIRIS Team MPS/UPD/LAM/IAA/SSO/INTA/UPM/DASP/IDA; J. Roger.
The European Space Agency (ESA) has been celebrating its Rosetta spacecraft, which was the first (and still only) craft to give us extremely close-up images of a comet. Rosetta ended its mission to comet 67P/Churyumov-Gerasimenko on September 30, 2016, with a controlled impact on the comet’s surface. Prior to that, it had monitored cometary evolution – in a way never seen before – for about a year before and after 67P came closest to the sun in August, 2015. On October 1, 2018, ESA released this image of the comet. Haunting, isn’t it? It shows a portion of the comet as viewed by Rosetta only one-and-a-half months after Rosetta began orbiting 67P in September, 2014. At the time of the image above, the spacecraft was just over 16 miles (26.2 km) from the comet’s surface. Amateur astronomer Jacint Roger Perez, from Spain, selected and processed this view by combining three images taken in different wavelengths by the OSIRIS narrow-angle camera on Rosetta. ESA said in a statement:
Seen in the center and left of the frame is Seth, one of the geological regions on the larger of the two comet lobes, which declines towards the smoother Hapi region on the comet’s ‘neck’ that connects the two lobes. The landscape in the background reveals hints of the Babi and Aker regions, both located on the large lobe of 67P/C-G …
The sharp profile in the lower part of the image shows the Aswan cliff, a 134 m-high scarp separating the Seth and Hapi regions. Observations performed by Rosetta not long before the comet’s perihelion, which took place on 13 August 2015, revealed that a chunk of this cliff had collapsed – a consequence of increased activity as the comet drew closer to the Sun along its orbit.

Before Rosetta, no one knew comets looked like this. Rosetta followed comet 67P/ Churyumov-Gerasimenko to its closest point to the sun and beyond. As the comet neared the sun, the spacecraft’s cameras saw jets erupting from comet. Image via ESA’s Rosetta spacecraft/ NASA.

As the images rolled in from Rosetta, scientists gave names to the various regions on the surface of 67P, to aid in their discussions. Image via Astronomy & Astrophysics.
ESA launched the Rosetta spacecraft in 2004. The craft took 10 Earth-years to reach the comet, ultimately making six orbits around the sun. Its journey included three Earth flybys, a Mars flyby, and two asteroid encounters. The craft endured 31 months in deep-space hibernation on the most distant leg of its journey, before waking up in January 2014 and finally arriving at the comet in August 2014.
By the way, ESA invites you to:
Explore the full mission image archive yourself at and let us know what hidden treasures you find via @esascience.

View larger. | Compilation of the brightest outbursts seen at Comet 67P/Churyumov–Gerasimenko by Rosetta spacecraft between July and September 2015, via ESA.
Bottom line: Amateur astronomer Jacint Roger Perez of Spain processed this Rosetta spacecraft view of comet 67P/Churyumov-Gerasimenko.
from EarthSky https://ift.tt/2NVQrEh
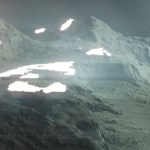

Comet 67P/Churyumov-Gerasimenko as seen by the Rosetta spacecraft on September 22, 2014. Amateur astronomer Jacint Roger Perez, from Spain, processed this image, which is via ESA/Rosetta/MPS for OSIRIS Team MPS/UPD/LAM/IAA/SSO/INTA/UPM/DASP/IDA; J. Roger.
The European Space Agency (ESA) has been celebrating its Rosetta spacecraft, which was the first (and still only) craft to give us extremely close-up images of a comet. Rosetta ended its mission to comet 67P/Churyumov-Gerasimenko on September 30, 2016, with a controlled impact on the comet’s surface. Prior to that, it had monitored cometary evolution – in a way never seen before – for about a year before and after 67P came closest to the sun in August, 2015. On October 1, 2018, ESA released this image of the comet. Haunting, isn’t it? It shows a portion of the comet as viewed by Rosetta only one-and-a-half months after Rosetta began orbiting 67P in September, 2014. At the time of the image above, the spacecraft was just over 16 miles (26.2 km) from the comet’s surface. Amateur astronomer Jacint Roger Perez, from Spain, selected and processed this view by combining three images taken in different wavelengths by the OSIRIS narrow-angle camera on Rosetta. ESA said in a statement:
Seen in the center and left of the frame is Seth, one of the geological regions on the larger of the two comet lobes, which declines towards the smoother Hapi region on the comet’s ‘neck’ that connects the two lobes. The landscape in the background reveals hints of the Babi and Aker regions, both located on the large lobe of 67P/C-G …
The sharp profile in the lower part of the image shows the Aswan cliff, a 134 m-high scarp separating the Seth and Hapi regions. Observations performed by Rosetta not long before the comet’s perihelion, which took place on 13 August 2015, revealed that a chunk of this cliff had collapsed – a consequence of increased activity as the comet drew closer to the Sun along its orbit.

Before Rosetta, no one knew comets looked like this. Rosetta followed comet 67P/ Churyumov-Gerasimenko to its closest point to the sun and beyond. As the comet neared the sun, the spacecraft’s cameras saw jets erupting from comet. Image via ESA’s Rosetta spacecraft/ NASA.

As the images rolled in from Rosetta, scientists gave names to the various regions on the surface of 67P, to aid in their discussions. Image via Astronomy & Astrophysics.
ESA launched the Rosetta spacecraft in 2004. The craft took 10 Earth-years to reach the comet, ultimately making six orbits around the sun. Its journey included three Earth flybys, a Mars flyby, and two asteroid encounters. The craft endured 31 months in deep-space hibernation on the most distant leg of its journey, before waking up in January 2014 and finally arriving at the comet in August 2014.
By the way, ESA invites you to:
Explore the full mission image archive yourself at and let us know what hidden treasures you find via @esascience.

View larger. | Compilation of the brightest outbursts seen at Comet 67P/Churyumov–Gerasimenko by Rosetta spacecraft between July and September 2015, via ESA.
Bottom line: Amateur astronomer Jacint Roger Perez of Spain processed this Rosetta spacecraft view of comet 67P/Churyumov-Gerasimenko.
from EarthSky https://ift.tt/2NVQrEh